Cancer Research
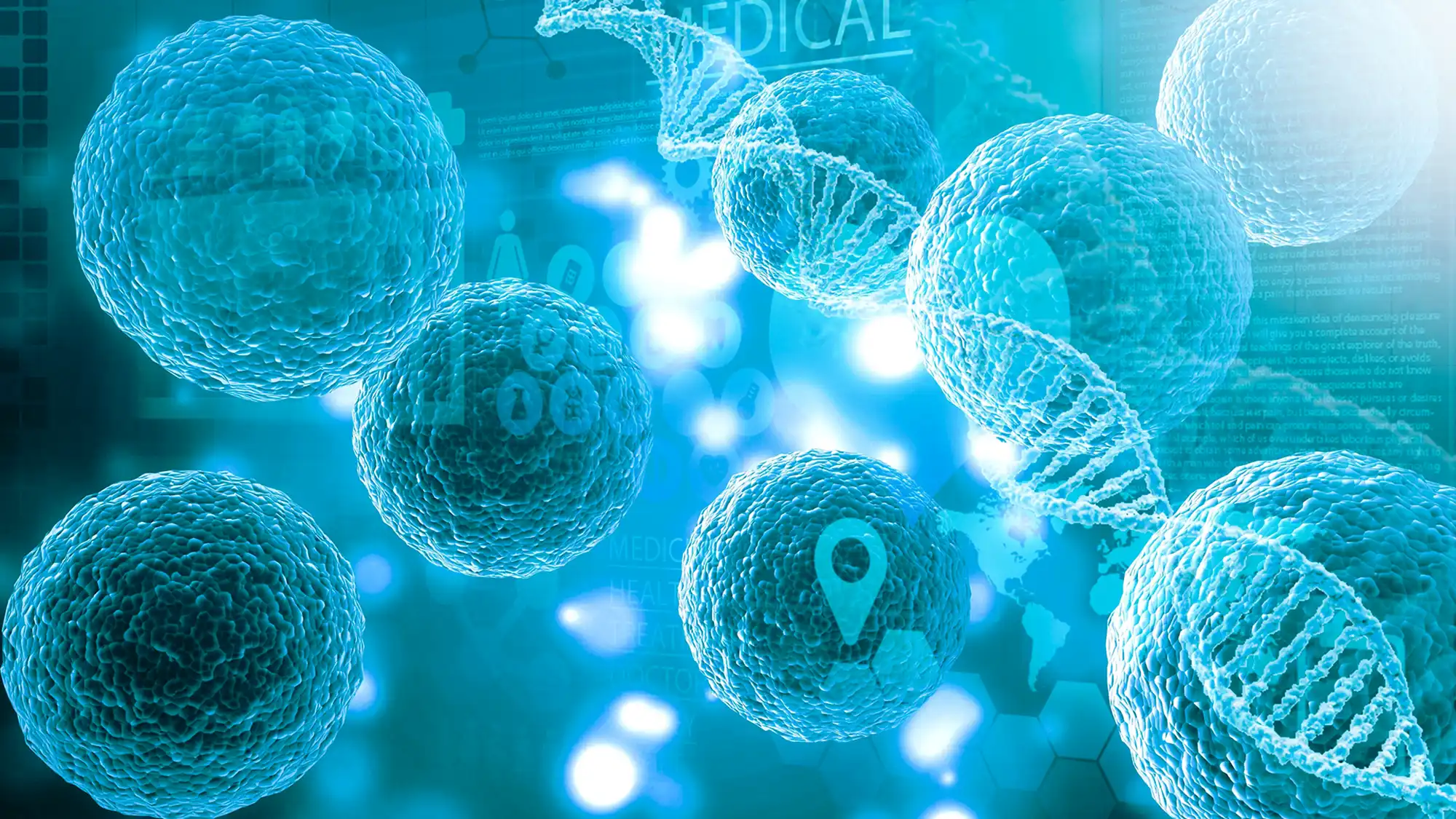
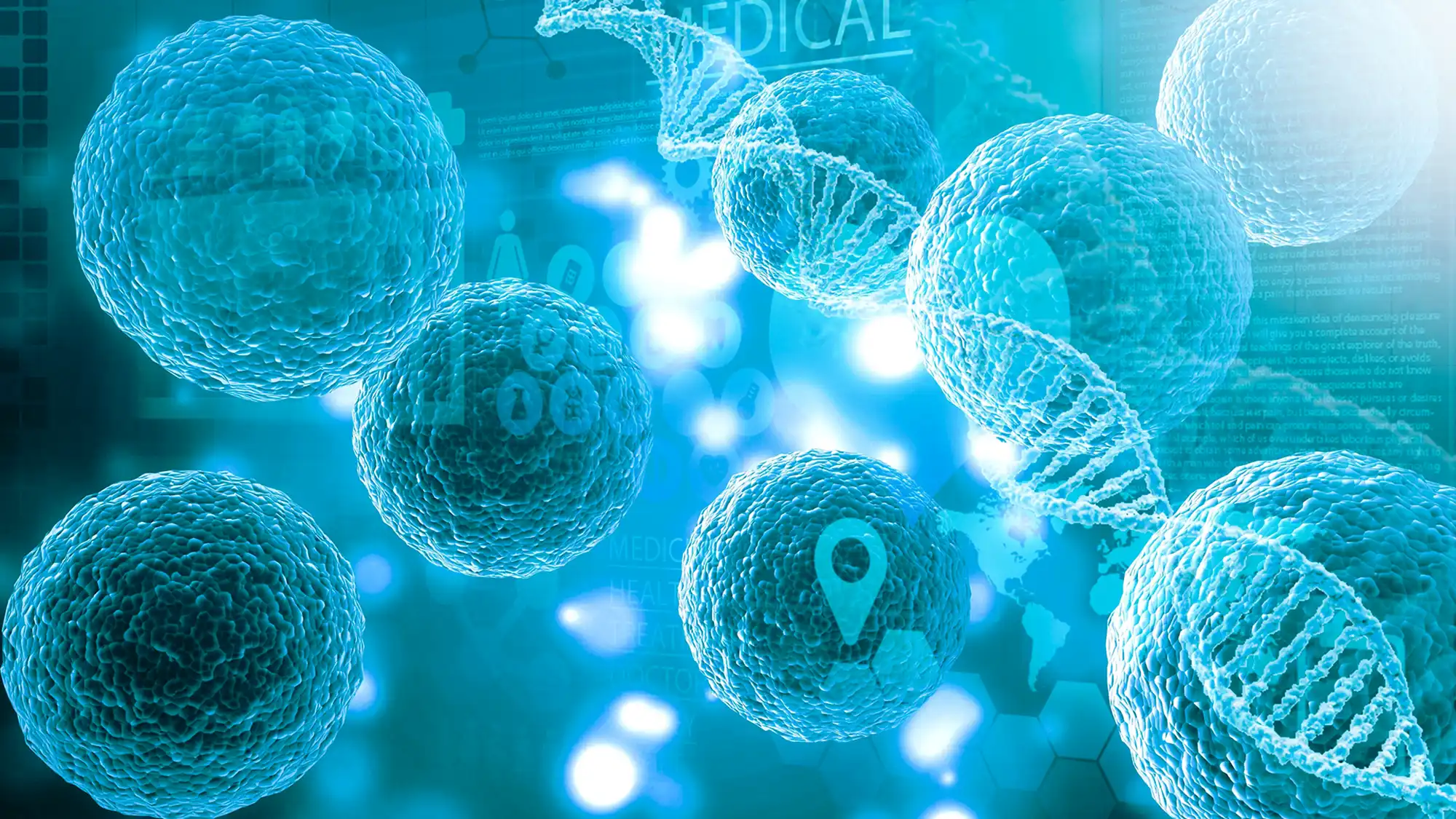
Genomic structural variations (SVs) encompass a diverse array of alterations involving large segments of the genome, such as deletions, duplications, inversions, translocations, and insertions. Many of these variations are most extreme in cancer cells where wholesale rearrangements such as chromothripsis can make genomic characterization via sequencing impossible1. These variations play a pivotal role in the initiation, progression, and heterogeneity of various cancers. Identifying the mechanism of how the rearrangements occur can often help to pinpoint the factors that underlie the cancer. Understanding and accurately characterizing SVs is crucial for comprehensive cancer research and patient care. High-performance electronic genome mapping provides a comprehensive view of genomic architecture, allowing precise identification of SVs, including complex and cryptic rearrangements, facilitating precise diagnosis, prognosis, and tailored therapeutic strategies.
Oncogenic Driver Identification
Identifying genomic SVs is fundamental for identifying some oncogenic drivers, which are critical mutations promoting cancer initiation and progression. Studies have shown that structural variations often disrupt key genes involved in cellular proliferation, differentiation, and DNA repair mechanisms2. Knowledge of these alterations aids in the selection of targeted therapies and the development of personalized treatment regimens for cancer patients.
Tumor Heterogeneity Assessment:
Cancer is characterized by substantial intra- and inter-tumor heterogeneity, influenced by genomic SVs3. This heterogeneity can make it difficult to create consensus maps with sequencing reads and therefore prevent the identification of breakpoints. By efficiently maximizing the detection of labels on ultra-long DNA molecules using electronic genome mapping, one can deconvolute complex breakpoint signatures and identify their precise positions. Accurate detection and characterization of breakpoints can enable the delineation of tumor heterogeneity, assisting in understanding clonal evolution and identifying potential therapeutic targets for different tumor subclones.
Enhanced Cancer Risk Assessment:
Germ-line SVs can significantly contribute to an individual’s cancer susceptibility and risk profile.4 Understanding the SV landscape associated with specific cancer types enables a more accurate assessment of cancer risk for individuals, aiding in proactive surveillance and risk management strategies. High-resolution electronic mapping can identify both germ-line and somatic SVs, providing valuable insights for comprehensive risk assessment4.
Early Detection and Diagnosis:
Early detection of cancer is pivotal for improving patient outcomes and survival rates. Genomic SVs often harbor diagnostic potential, serving as early markers for specific cancer types. Integrating electronic mapping tools into clinical workflows allows for the precise detection of SVs at an early stage, facilitating timely and accurate diagnosis4. Early intervention based on SV profiling contributes to more effective treatment strategies.
Personalized Targeted Therapies:
Genomic SVs frequently disrupt key genes and signaling pathways involved in cancer progression, making them potential therapeutic targets5. Accurate identification and annotation of these SVs using advanced genome mapping technologies provide a basis for tailoring targeted therapies, improving treatment response, and minimizing adverse effects6. Precision medicine approaches guided by SV profiling are increasingly shaping cancer treatment paradigms.
Predicting Drug Resistance:
Resistance acquired over time to targeted therapies is a major challenge in cancer treatment. Genomic SVs can confer resistance to therapies by altering drug targets or affecting signaling pathways. Understanding these alterations through precise SV detection can lead to more effective therapeutic options and improving treatment outcomes7.
- Sidiropoulos N, et al. Somatic structural variant formation is guided by and influences genome architecture. Genome Res. 2022; 32:643-655. doi: 10.1101/gr.275790.121.
- Zhang M, et al. Inferring Homologous Recombination Deficiency of Ovarian Cancer From the Landscape of Copy Number Variation at Subchromosomal and Genetic Resolutions. Front Oncol. 2021; 11:772604. doi: 10.3389/fonc.2021.772604.
- Sobral D, et al. Genetic and microenvironmental intra-tumor heterogeneity impacts colorectal cancer evolution and metastatic development. Commun Biol. 2022; 5:937. doi: 10.1038/s42003-022-03884-x.
- Davidson AL, et al. The clinical utility and costs of whole-genome sequencing to detect cancer susceptibility variants-a multi-site prospective cohort study. Genome Med. 2023; 15:74. doi: 10.1186/s13073-023-01223-1. .
- Paulson TG, et al. Somatic whole genome dynamics of precancer in Barrett’s esophagus reveals features associated with disease progression. Nat Commun. 2022; 13:2300. doi: 10.1038/s41467-022-29767-7. .
- Zhang W, et al. Non-invasive plasma testing for CD274 UTR structural variations by next-generation sequencing in cancer. Cell Death Discov. 2023; 9:35. doi: 10.1038/s41420-023-01316-1.
- Kobayashi Y, et al. Genomic and biological study of fusion genes as resistance mechanisms to EGFR inhibitors. Nat Commun. 2022; 13:5614. doi: 10.1038/s41467-022-33210-2.
Our Products
The state-of-the-art OhmX Platform uses electronic nano-detectors to deliver the highest resolution for whole genome structural variant analysis. You can now perform whole genome analysis of SVs down to 300bp in size—enabling insights into previously undetectable DNA variations.
Learn More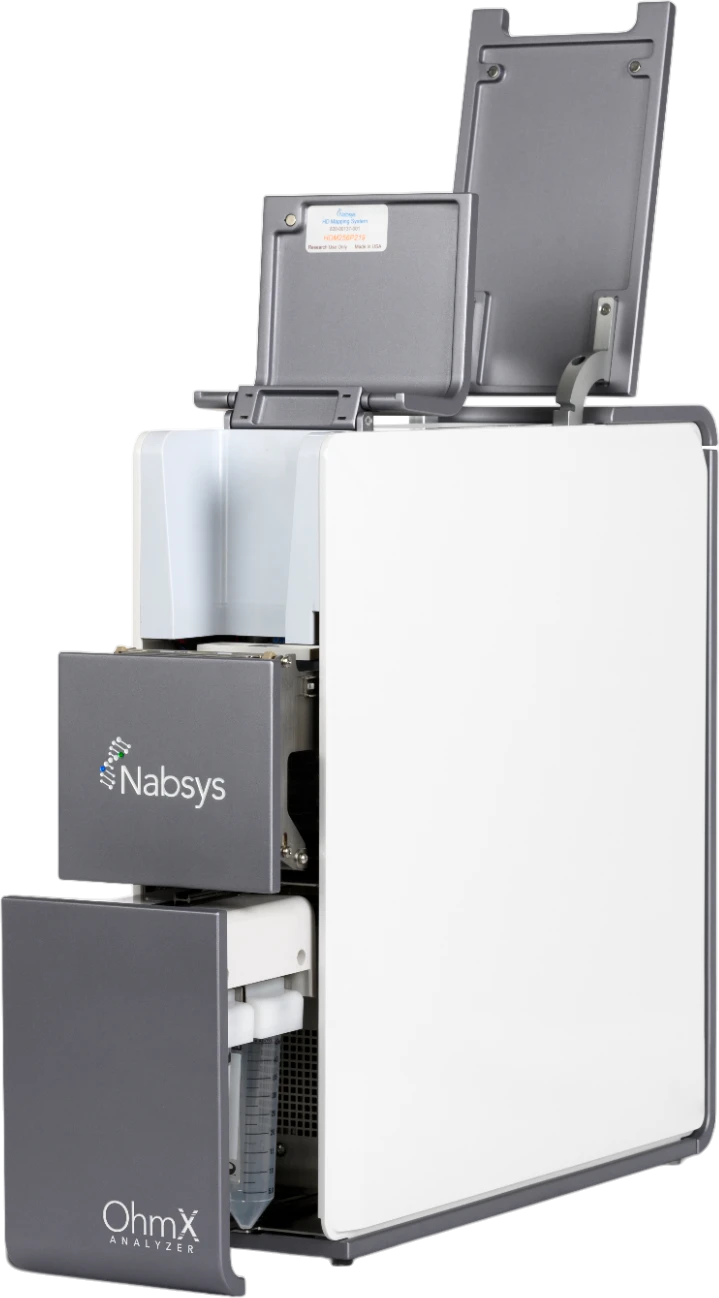
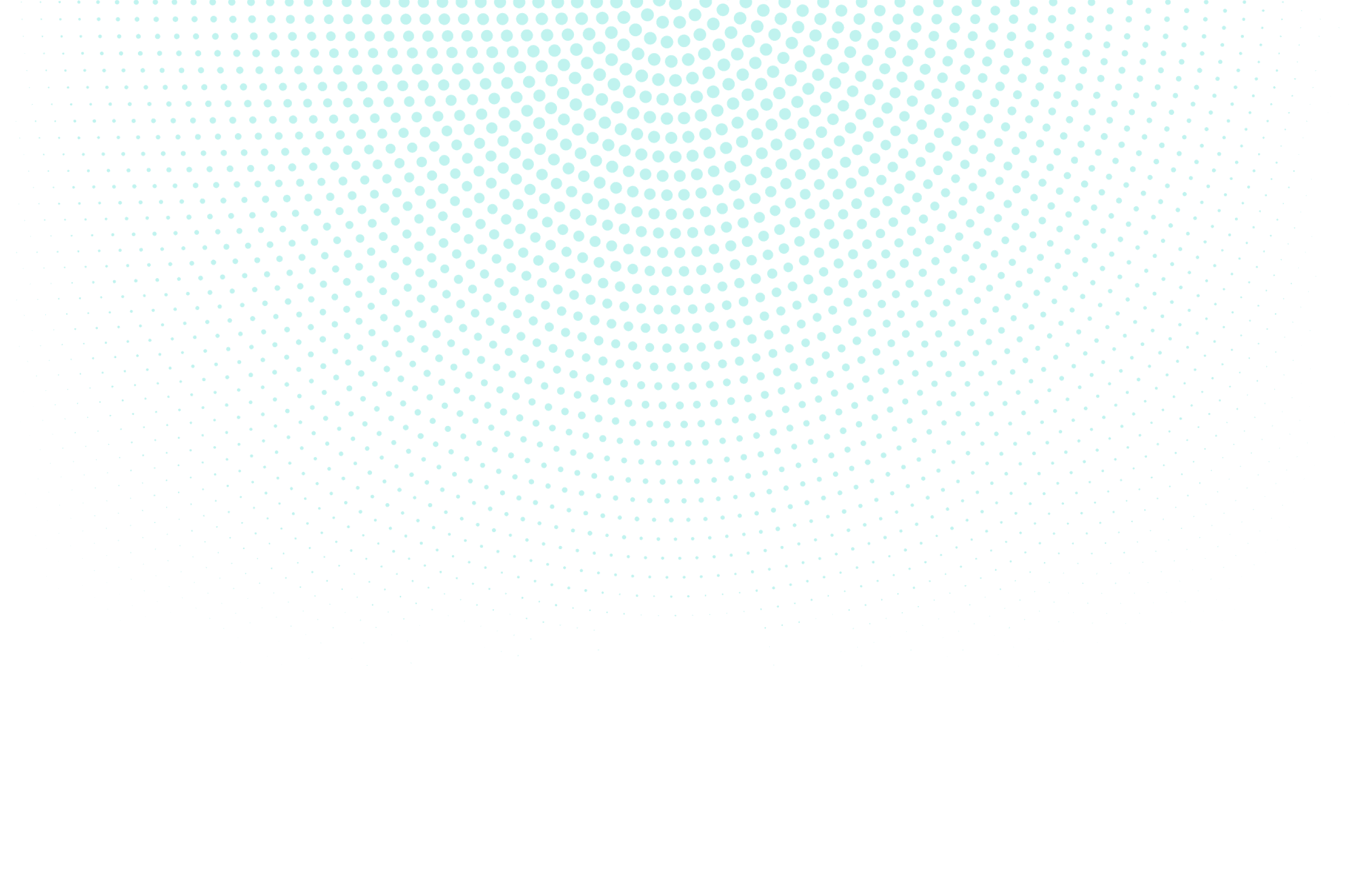